Marvel comics creator Stan Lee said that the inspiration for the Spider-Man character came in part from watching a spider climbing a wall. But Lee himself admitted he was unsure whether the story was real or whether he had invented it, and his colleagues Jack Kirby and Joe Simon disputed his authorship of the character. It is not unusual for an immortal creation to have several originators, especially when in reality the idea seems so simple that it could have occurred to anyone: a human with the powers of a spider. However, outside the silver screen and the world of comic books, emulating in our real world the powers that spiders have gained over 400 million years of evolution is a much more complicated thing, and one that science has been pursuing for years.
Humans have been using silk from silkworms for thousands of years, and some indigenous communities have learned how to exploit spider webs: Australian Aborigines harvested them for thread and fishing nets, and there are reports of them being woven in some places. But when the French government commissioned the naturalist Rene-Antoine Ferchault de Reaumur to obtain spider silk in 1709, it was soon revealed that the task was not so easy. He attempted to collect it from egg sacs for the purpose of making stockings and gloves, but obtained very little. His compatriot François Xavier Bon de Saint Hilaire tried to breed spiders for this purpose, but discovered that it was complicated: in captivity spiders tend to eat each other, and must be immobilised to obtain the silk. In 1829, the British inventor Daniel Bransdon Rolt invented an ingenious but complex apparatus consisting of a steam engine and a bobbin that collected silk from an individual spider; he managed to harvest thousands of metres of thread.
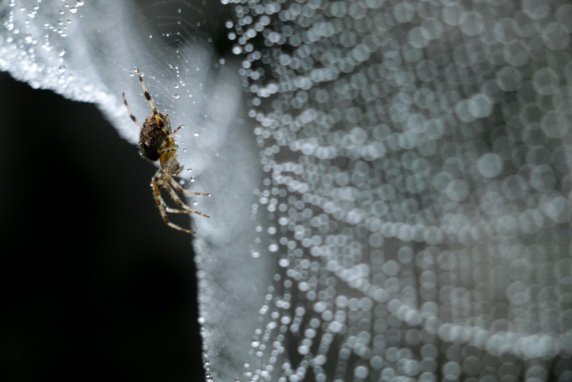
But this and other historical attempts, with varying degrees of success, encountered the same problems, leading to a single conclusion: large-scale industrial exploitation of spider silk was unfeasible. And yet, as the prodigious properties of this material were discovered in detail, there was growing interest in this material of similar strength to steel (much greater for the same mass), but enormously elastic and light; a thread that could circle the globe would weigh less than half a kilo.
In addition, spider silks are temperature resistant, can conduct electrical current, inhibit bacterial growth and are virtually invisible to the human immune system, suggesting possible medical applications. For all these reasons, they are one of the great promises of the new materials. Unfortunately, the difficulty of obtaining spider silk originally restricted it to a few very specific uses, such as the sight lines of telescopic weapons and optical instruments, where a fibre much finer than a hair was needed.
It was with the development of molecular biology and modern laboratory instruments that the goal of exploiting the properties of spider silk was revived. But the aim is no longer to obtain it from nature, but to manufacture it. This is no easy task either, as it is a fibre made of proteins of enormous size. But even having identified these proteins, it is much more complicated that just producing them in the laboratory; spider silk is liquid in the animal’s glands and solidifies when released, but it does so with a specific configuration and orientation without which those coveted properties do not exist.
The ‘secret’ genes of spiders
Of the more than 50,000 known spider species, all of which produce silk, research on this material has traditionally focused on the golden silk orb-weaver spider (Trichonephila clavipes or Nephila clavipes), a tropical species indigenous to the Americas. In 2017, a team of researchers led by the University of Pennsylvania (US) sequenced its genome, almost as large as that of humans, in which more than 14,000 genes were identified; 28 of them are responsible for producing the silk proteins or spidroins, joined by another 649 genes that are also expressed in the silk glands and likely play roles in silk solidification and the structure of the fibres.
“It is perhaps the resilience, the combination of strength and flexibility before a thread breaks, which is of greatest interest to the scientific community in general,” Benjamin Voight, director of the sequencing study, tells OpenMind. However, the large number of genes involved and their different combinations of expression in the glands have surprised the researchers, who did not expect such complexity in the silk-making process. Understanding it in minute detail is essential to imitate it in the laboratory. “We must learn what sequences generate diversity in the biophysical properties of silk: tensile strength, stiffness, stretchability, durability or adhesion,” says Voight.
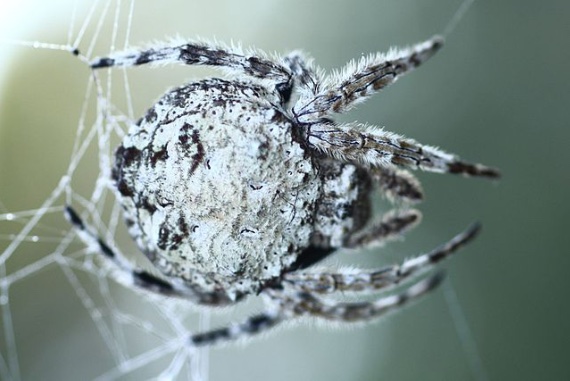
More recently, Voight’s and other research groups have analysed the genome of another species, Darwin’s bark spider (Caerostris darwini), discovered in 2010 in Madagascar. It produces silk that is more than twice as resilient as that of other spiders and 10 times tougher than Kevlar, which is used in bulletproof vests; it is the toughest biological material known. In its natural habitat, strands up to 25 metres long have been observed stretched between the two banks of a river.
The results show that this spider has an even larger genetic repertoire of spidroins than Trichonephila clavipes: at least 31 genes, including new types of sequences absent in other species, and whose produced proteins mix in the glands in a way that is also more complicated than previously observed. What’s more, the spinning ducts of their glands are unusually long, which may facilitate the alignment of proteins into extremely tough fibres. According to the researchers, all this complexity holds the secrets to the extraordinary toughness of the silk, but much remains to be unravelled.
From shampoos to microsutures
Turning spider web into a commercial product also involves unlocking the unique structure that liquid silk acquires as it solidifies. In the 1960s, researchers began to analyse it using X-ray diffraction techniques, and in the 1990s the use of Nuclear Magnetic Resonance (NMR) revealed more details about its crystalline components. Recent new studies using different types of spectroscopy have observed the structure of the nanofibrils that make up the silk at the molecular scale in unprecedented detail.
Finally, a robust production method needs to be found. “Private companies have techniques to do this and are looking for sequences and materials with optimal properties for their production on an industrial scale,” says Voight. Chemical synthesis is not enough, so researchers are turning to genetic engineering, introducing the genes for the spidroins into another organism that will make the proteins from which silk can be artificially produced. For this task, the most classic resource is the bacterium Escherichia coli, although transgenic goats, yeast and plants have also been tried. These are the options chosen by Randy Lewis of Utah State University, who cloned the first spider silk gene in 1990 and has been pursuing the elusive goal of recreating real spider webs ever since. Recently, thanks to the new CRISPR gene-editing technology, Lewis and his team have succeeded in obtaining transgenic silkworms that produce spider silk-like fibres.
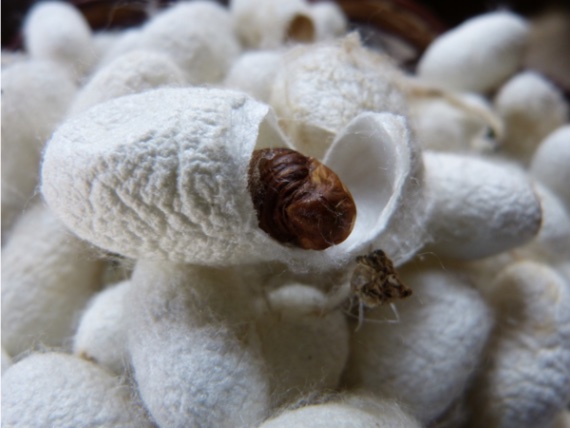
“There are several companies that claim to produce spider silk, but in all cases the fibres they are producing are not real proteins but rather computer-designed simulations,” Lewis tells OpenMind. We may still have to wait for spider silk to become a mass product, but experts predict that it may not be far off. In fact, these simpler versions of silk, with smaller-sized proteins, are already being applied to a variety of tentative uses: prototype garments such as an Adidas running shoe or a lightweight parka by The North Face, or cosmetics and shampoos that take advantage of the silky, moisturising qualities of these proteins. Of particular interest are medical uses, such as coatings for prostheses to prevent their rejection by the immune system, sutures or matrices for transplant tissues grown in vitro.
And who knows what else: “When I say that we’d like to build a ‘web-shooter’ like Spider-Man’s in the lab, I’m only half joking,” said Voight.
Comments on this publication