3D bioprinting is the use of 3D printing technology to create functional biological tissues through the precise and controlled, layer-by-layer 3D patterning of biogels composed of different types of cells, structural (bio)polymers, growth factors and other biomolecules. But 3D bioprinting is much more than that. It is science fiction (about to become) reality.
The scenario is shifting from one of high demand for organs for transplant patients to one where replacement organs and tissues could be produced on demand for anyone in need. And these organs would be made from the patient’s own cells, so there would be no problems with rejection. This is a scenario for which experts have set a realistic deadline of around 10 years, the time they believe is needed to overcome the difficulties and limitations that this revolutionary technique still faces today.
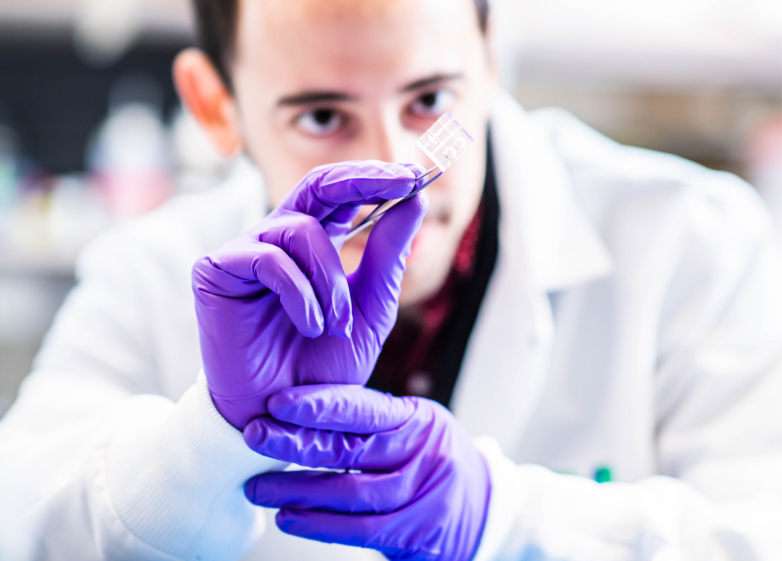
But even as they work towards this milestone, doctors and researchers are already beginning to harness the enormous potential of 3D bioprinting in other areas of medicine, such as the study of pathologies, the testing of new drugs and treatments, and cancer research.
A mini-heart for pumping out research
One of the most immediate applications with the greatest potential—so much so, in fact, that it is already being put into practice—is the bioprinting of mini-organs and tissues. These are miniaturised functional units printed inside fluid-filled sacs that replicate the conditions inside the organism. The process makes it possible to print collections of these mini-tissues on a single support to study pathologies in depth, and to test drugs and treatments much more quickly and directly than in traditional clinical trials.
Following this approach, a multidisciplinary group of researchers at Boston University has created a miniature replica of a human ventricle—one of the four chambers that make up the heart, and the one most involved in pumping blood—with the capacity to beat, by bioprinting an acrylic shell that is rigid yet flexible enough to support the layers of living heart cells deposited on it, and to support the heartbeat they generate. The miniPUMP, as it has been dubbed, occupies a surface area of just 3cm2, little more than a postage stamp, and is beginning to be used to study how the heart grows and develops in the embryo, how different pathologies affect it and, above all, as a test subject to study the efficacy and side effects of potential new treatments.
Seeing is believing
Today, perhaps the greatest limitation to overcome in 3D bioprinting of fully functional organs is achieving proper vascularisation: both internally, to ensure adequate exchange of nutrients and waste products, and externally, to connect with the body’s blood vessels and integrate into the circulatory system. This limitation is minimised in very poorly vascularised or avascular tissues, which lack blood vessels, such as cartilage or the cornea—the outermost layer of the eye—and these are the areas where most progress has been made.
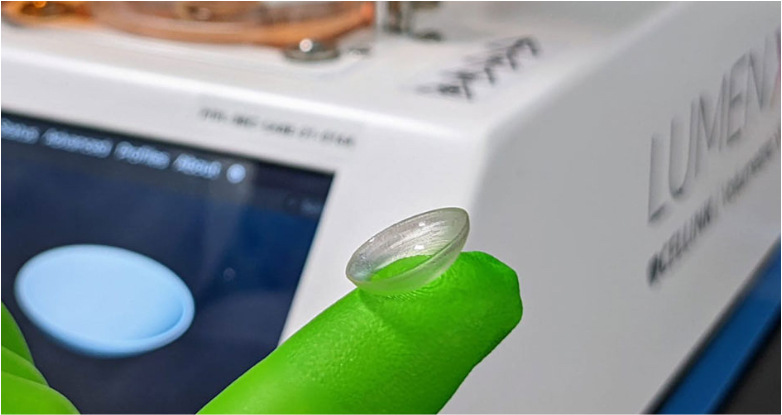
In 2021, for example, researchers at Stanford University succeeded in 3D printing human corneas in the laboratory, which are now being tested as replacements for patients suffering from corneal blindness.
Replacement ears
In the same vein, other organs and tissues that are currently viable for 3D bioprinting are external cartilaginous tissues, such as the nose and ears, due to their minimal vascularisation and the ease with which they can be connected to the organism because they are located “outside” the body. In June 2022, the first transplant of a bioprinted ear was performed on a 20-year-old woman suffering from microtia—a congenital problem in which the auricle, or external part of the ear—is underdeveloped, reducing the ability to hear. The ear was created from a sample of chondrocytes—cells that form cartilage—taken from the patient, which were cultured to replicate and provide the starting material for printing the tissue. It was then implanted under the skin, where the regeneration of the cartilage was completed with the formation of a bridge between the implant and the original tissue.

Meniscus printed in space
Another limitation of current 3D bioprinting is ensuring that the biogel layers do not collapse under their own weight during printing before they can fuse together and solidify. To overcome this problem, one option—the most common—is to print the tissue on a rigid scaffold, either synthetic in nature or, where possible, made from the organism’s own biopolymers. But another, less explored, option is bioprinting under microgravity conditions. This is precisely the method chosen by the company Redwire Space, which successfully 3D printed a life-size human meniscus—an avascular piece of cartilage in the knee joint—in the laboratory of the International Space Station. Once the meniscus was printed and cultured in space, it was shipped back to Earth to study its functionality and viability as a replacement for deteriorated originals.
The skin we live in
As we have already seen, one of the most advanced areas in the use of 3D bioprinting is regenerative medicine, specifically the replacement of damaged or mutilated external tissues or parts of tissues. Working in this area, the French company Poietis created its first bioprinted human skin model in 2018, made from human fibroblasts embedded in a collagen matrix and covered by a layer of keratinocytes. In 2020, the company went a step further and installed its first bioprinting platform in a hospital to begin clinical trials of grafts in patients with severe burns. In parallel, the company continues to develop increasingly successful skin models. These skin replicas have been and continue to be used by pharmaceutical and cosmetic companies to test their new products.

Tumours trapped in biogel
Another area of medicine in which bioprinting has enormous potential for application is cancer research. The difficulty in finding suitable treatments, as well as the resistance of tumours to existing treatments, is linked to their heterogeneous cellular nature—that is, tumour cells are different from normal cells and behave differently. Therefore, studying the interactions of tumour cells with each other and with their microenvironment, is key to understanding the progression of cancer.
This is the reason why successes such as that announced a few days ago by an international team of researchers are so significant. The team succeeded in creating in vitro models of breast cancer tumours by bioprinting from human tumour and epithelial cells embedded in biogel that reproduces the microenvironment in which these tumour cells develop. With this success, the researchers hope to discover which factors favour their multiplication and thus develop more effective treatments.
Miguel Barral
Comments on this publication