Who would have imagined that the new genetic revolution of the 21st century would be born in a pond designed to harvest salt from seawater? In the salt flats of Santa Pola (Spain), the study of a microbe led to the discovery of the CRISPR/Cas9 system, the best tool existing today to cut and paste DNA fragments and the great promise of gene therapy for this century.
None of this was in the mind of University of Alicante microbiologist Francisco Martínez when, in 1990, he was studying why salt concentration affected the way in which an archaeon (simple unicellular organisms other than bacteria) from those salt flats, the so-called Haloferax mediterranei, responded differently to restriction enzymes, which cut DNA at specific sequences and are common tools in every molecular biology laboratory.
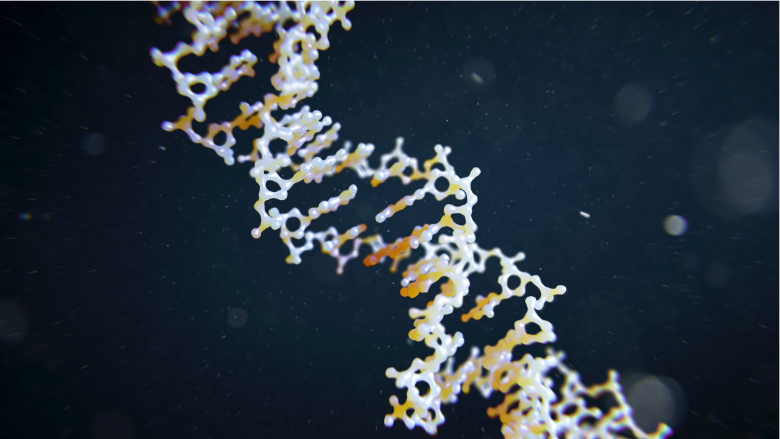
When looking for answers in the genome of this and other archaea, Mojica observed some DNA sequences that were repeated, separated by heterogeneous spacers. The intrigue over these sequences increased when Mojica discovered similar ones in a score of different microbes, to which were added other cases found by different researchers. In 2002, Mojica suggested a name for these mysterious pieces of repeated DNA: CRISPR, or Clustered Regularly Interspaced Short Palindromic Repeats. The genes close to these sequences, which were assumed to be related to them, were called genes associated with CRISPR, or Cas. The CRISPR/Cas system now had a name, but its function was still a mystery.
It was then that Mojica had the brilliant intuition that would lead him to solve the enigma. Instead of focusing on what all those sequences had in common, the repetitions, he decided to look at what differentiated them, the spacers. When studying one of them in the bacterium Escherichia coli, he discovered that it was identical to a segment of the genome of a virus called phage P1 that infects this bacterium. Or that should infect it, since that E. coli specifically was immune to P1.
Sequences of infective DNA
Following this promising thread, the researcher checked the spacers of thousands of other CRISPRs in different microbes, noting that in all cases they corresponded to sequences of infective DNA, but that they always appeared in bacteria immune to those infections. Thus, Mojica proposed a plausible, but risky hypothesis: that the CRISPRs represented an adaptive immune system of the bacteria that collected pieces of DNA from its attackers to recognize them in the future and eliminate them.
So daring was the idea that Mojica’s study was rejected without review by the journal Nature, and later by others, until in 2005, after a long process of corrections, it was published in the Journal of Molecular Evolution. Shortly after, French researcher Gilles Vergnaud and his collaborators proposed a similar hypothesis. But the bacteria’s immune system was still an exclusive concern of microbiologists and CRISPR/Cas was still far from launching the genomic editing revolution.
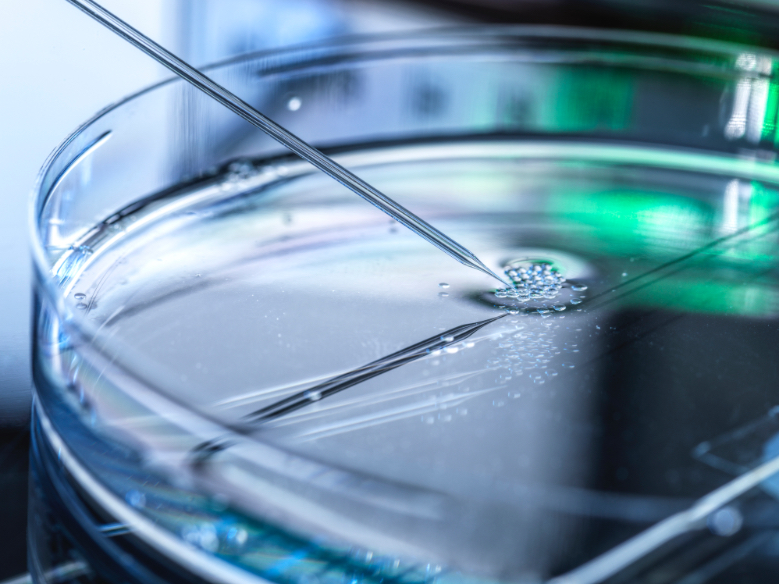
The breakthrough came when it became known how bacteria use the CRISPR/Cas system to rid themselves of viruses. Cas genes make enzymes that cut DNA, while CRISPR spacers produce RNA molecules capable of recognizing their twin sequences in the viral genome. The RNA and the Cas enzymes travel together, so that the former act as a guide to indicate to the latter where they must cut to disable the virus.
Realising that these molecular scissors could be modified to cut any DNA sequence, American biochemist Jennifer Doudna and French researcher Emmanuelle Charpentier simplified and redesigned the CRISPR system of the bacterium Streptococcus pyogenes to build the CRISPR/Cas9 tool, which was later optimised and tested in human cells by researchers Feng Zhang and George Church. The system is completed with a fragment of DNA that acts as a mould to repair the broken ends and that allows for the insertion of the desired sequence, for example the correct version to restore a gene. The cellular repair machinery itself handles the final bonding. In 2020, Doudna and Charpentier were awarded the Nobel Prize in Chemistry, an honour that Mojica and other pioneers did not receive due to the restrictive rules governing these awards.
A system with great advantages and some limitations
CRISPR/Cas9 was not the first gene-cutting tool available, but it has major advantages over other systems: “Basically, the ease with which you can program the system to direct it to the target where you want it to act,” Mojica told OpenMind. “With the other tools, you have to introduce multiple modifications to the protein sequence, which is tedious and expensive, whereas with CRISPR you just have to synthesise a small RNA guide molecule, which is cheap and easy to design, and use the Cas9 protein as it is. In addition, the researcher adds, CRISPR/Cas9 makes it possible to target several genes at the same time.
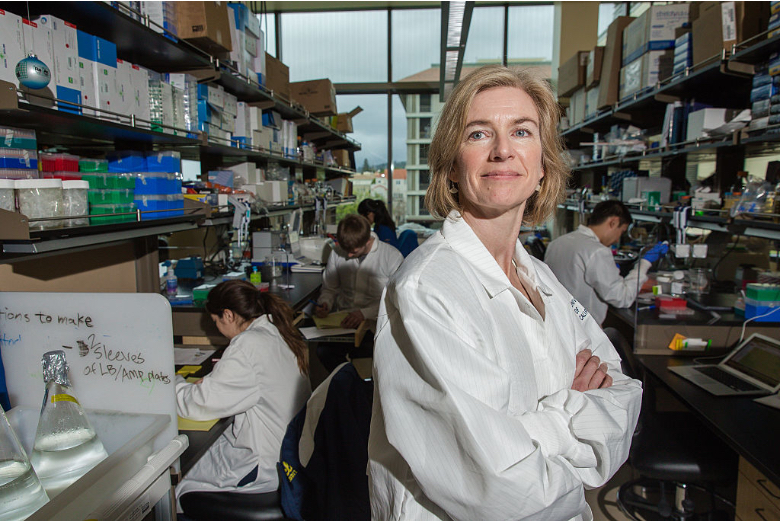
Over the years, new modifications and versions have been added to the original system, thanks to the introduction of different Cas, so that today there are two classes divided into a total of six types, which in turn are divided into 33 subtypes. The two classes differ in whether Cas is a protein complex or a single protein, and the different types target DNA, RNA or both. In 2019, a variant was created that incorporates several techniques, known as prime editing, to precisely search for and alter sequences. All these advances have taken CRISPR out of the research laboratory, where it has been an immensely useful tool since its inception, and into the practical uses it was predicted to have.
These include the creation of genetically modified crops as an alternative technology to transgenics, since in this case it is possible to edit existing genes directly, without the need to introduce transgenes (genes from another species or marker genes) unless one wishes to do so. Given the unpopularity of transgenic crops and the legal obstacles to their further expansion, CRISPR should facilitate the adoption of these improved crops because they are not transgenic. However, regulation is not yet final or unanimous; for example, the EU still treats CRISPR crops in the same way as transgenic crops. Whether this will change in the future remains to be seen.
The applications of CRISPR also extend to gene therapy. In theory, it will be possible to correct defective genes that cause congenital diseases, but also to boost immune system functions to fight cancers, to disable genetic variants that put people at risk of certain diseases, to modify cells to restore lost functions—for example in diabetes—or to produce organs suitable for xenotransplantation (from other species) to avoid rejection.
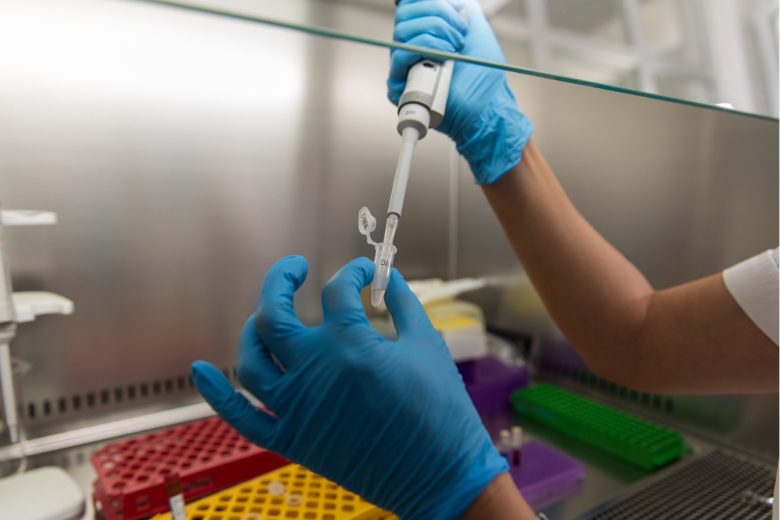
Depending on the case, gene therapy can be applied directly in vivo, in the patient’s body, or ex vivo, in cells removed from the patient’s body and then returned to the body. In 2023, the first drug using CRISPR was approved in Europe and the US for the ex vivo treatment of two blood disorders—sickle cell disease and beta thalassaemia—and numerous clinical trials are currently underway for other applications, including the use of CRISPR in vivo.
The next few years will undoubtedly see an explosion of practical applications for CRISPR, but also a growing debate about its limitations. In 2018, Chinese scientist He Jiankui announced the birth of two babies whose genes had been edited to confer resistance to the AIDS virus. Along with other experts who questioned the validity of the procedure for its intended purpose, the experiment was widely condemned by the scientific community, and He was jailed in his country. In addition to the ethical objections that some of the potential uses of CRISPR may raise, the technology will need to be refined to ensure that it works as and where it should, without failing due to over- or under-performance. Any revolution is a work in progress.
Comments on this publication