For many centuries, it was considered that there were only three states of matter: solid, liquid and gas (the three that are present and stable in our world). And water is the substance that best represents them, because it is the only one that exists naturally in the three states.
But the development of new technologies able to produce more and more extreme and energetic conditions in the laboratories has meant that in recent years five other states have been discovered (plus a final candidate that has just appeared in 2018).
Plasma
The plasma state is the one in which the gases contained inside neon lights, fluorescent tubes and, of course, plasma screen TVs exist. It is also the state that characterizes the aurora borealis and lightning. In fact, it is estimated that 99% of the matter in the observable universe is plasma.

Plasma is an ionized gas at a high temperature. Formed by cations (molecules or atoms with a positive charge) and free electrons (with a negative charge), between which significant electrostatic forces are present. Although as a whole the total electric charge is zero, in its interior the particles have a charge. This means that, unlike gases, plasmas are conductors of electricity and can be confined inside magnetic fields.
Quark-gluon plasma (QGP)
This is the state of matter in which (it is assumed) all the matter of the universe was just one millionth of a second after the Big Bang and just before beginning to cool down and change to other less energetic states. At that moment—characterized by extreme temperature and energy—all matter would be found as a dense soup of fundamental particles: quarks and gluons, moving at speeds close to that of light. Thus, the attractive forces between them are so weak that each one can maintain its individuality and move freely.
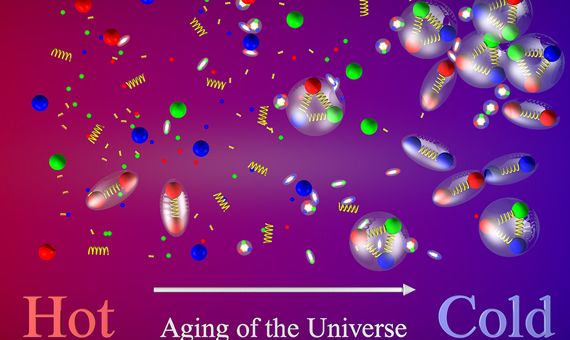
The first evidence of the existence of the QGP state, also known as quark soup, was uncovered in 2003 and confirmed in 2005 at the CERN accelerators. There it was found that quark-gluon plasma did not behave like an ideal gas (as was presumed) but rather as a superfluid, with minimum viscosity. So far, QGP has only been obtained in very specific installations and for a very limited time, and its applications are still being studied.
Quantum liquid spins
Physicist and Nobel Prize winner Philip Warren Anderson was the first to predict the existence of quantum liquid spins in the 1970s, but it was not until 2016 that their actual existence was proved. The curious thing is that, under certain conditions of pressure and temperature, some minerals exhibit regions in this state, among them, herbersmithite.

Spin is a property of electrons and other subatomic particles. Put simply, it’s as if each electron contained a tiny internal compass. In most materials (and in the states of matter) the spins of the electrons are aligned with each other. However, in the quantum liquid spin state, the spins of electrons never come to align, but remain in a constant fluctuation even at temperatures close to absolute zero, while in the remaining states of matter the spin freezes at that temperature.
The quantum liquid spin state gives the material unique magnetic characteristics, whose applications are currently being investigated.
Degenerate state
Under extreme pressures, such as those that occur within the nucleus of some stars, the particles are compressed into a minimum space. Since two particles cannot occupy the same space at the same moment, this causes the atoms to degenerate and lose their structure: the electrons leave their orbits and begin to move at speeds closer and closer to that of light, exerting an expansive force that compensates for the external pressure.

If this continues to increase and exceeds the so-called Chandrasekar limit, then the external pressure becomes untenable and the atomic nuclei also degenerate, losing their structure, collapsing into an accumulation of neutrons and protons.
Bose-Einstein condensate
In 1924, Satyendra Bose and Albert Einstein predicted the existence of a new state of matter by applying statistics to quantum mechanics. According to the two physicists, when matter cools to temperatures just above absolute zero, in some cases the particles that constitute it all fall to the same low energy level or quantum state. This situation violates the principles of quantum physics: the particles become indistinguishable from one another and form a sort of “super-atom.”

But it was not until 1995 that Cornell, Wieman and Ketterle managed to produce a Bose-Einstein condensate thanks to the use of new and more powerful lasers and electromagnets. Since then, it has been proven that this state is characterized by superfluidity and superconductivity, and also why it is capable of slowing down the speed of light, which passes through it at speeds of just a few metres per second.
Superionic ice
Water as a beginning and as an end. Water is the only substance present in nature in the three classical states, and it is also the substance in which, at the beginning of 2018, a new form or state of arrangement was discovered: superionic ice. To achieve this, ice crystals were subjected to a pressure 2 million times higher than atmospheric pressure and at a temperature close to 5,000°C. That brutal pressure forces the ice to adopt a very compact packing, but, at the same time, the high temperature dissolves the bonds of the water molecule. The result is that in superionic ice two phases coexist: one liquid and one solid. Oxygen atoms adopt a crystalline structure, through which hydrogen nuclei flow.

It is believed that superionic ice could exist in large quantities in gaseous and icy giant planets such as Uranus or Neptune, within which the appropriate conditions for its formation are found. If it were confirmed that other substances subject to similar conditions also adopt this arrangement, we would be in the presence of a new state of matter.
Miguel Barral
Comments on this publication